The face of sulfur is like that of chemistry. It finds its way into compounds that are poisonous and/or foul-smelling to us, making it easy for us to conjure a negative but starkly incomplete image of sulfur. In the bigger picture, however, there would be no life and less rain without its compounds. If sulfur existed, but if we had never learned how to modify the elemental form that occurs naturally, our world would be radically different.
Orthorhombic sulfur (S8) is the most common of several possible, so-called allotropes, molecular combinations involving a single element. It is one of the few elements known to the ancients because it can occur unbonded to other elements near volcanoes and hot springs. Egyptians used it on wheat 2000 years ago to prevent rust disease.
The element is bright yellow, and if you handle it to feel its smooth texture, you will walk away with smelly hands. When I was young, a kid in our neighbourhood, François, invited me to take a whiff of a sample of burning sulfur. (With a match, he had lit a sample from his chemistry set.) It turned to an attractive blue colour. The unusual flame incited me to move closer, but the smell felt like needles going up my nostrils. Of course, François laughed at my reaction. When I saw it again months later in my brother’s chemistry set and in school, I made sure not to burn it with my nose nearby; yet the indelible mark it left did not overcome the appeal of its distinguishing color.
i. Sulfur from tar sands
Since childhood I have encountered elemental sulfur countless times, even using it to clean up spills of mercury when it was still allowed in high school labs. Not once did I wonder about its origins until recently when I saw hills of sulfur at the port in Vancouver(Figure 1). It has apparently been a common sight for a long time, and it comes from Alberta’s tar sands.

There is a fair amount of sulfur in their heavy crude. In the refining process poisonous hydrogen sulfide, H2S, is produced, which is absorbed and separated from hydrocarbon gases by an alkaline (basic)compound. The sulfur is finally extracted by the Claus Process, which consists of a two-step-process: high- temperature oxidation of H2S and conversion to sulfur with a catalyst. A lot of sulfur is extracted this way, more than can be sold, and with the excess they decided to build pyramids out of pure sulfur, just outside of Fort McMurray, Alberta (Figure 2).

The process does not remove all sulfur, which becomes a problem because burning heavy crude produces not only carbon dioxide but sulfur dioxide. From 2018 onwards, global regulators were trying to crack down on sulfur dioxide emissions, which could shrink the market for heavy crude and force Canadian oil sands companies to further discount their product.
What happens to sulfur that’s sold and not used to make pyramids? Its most common destiny is sulfuric acid, to be explored in the next section. Sulfur can also be used to make matches, dandruff shampoo and fertilizer. In the elemental state, it is used as a fungicide in organic or industrial farming. Although there are “sulfur-shy” plants such as gooseberries, apricots and raspberries that should never be treated with sulfur, others tolerate the element, which can prevent powdery mildew, rose black spot and fungal rust. Since sulfur is a preventive fungicide it will not work if the diseases have already appeared.
ii. Sulfur from photosynthetic bacteria
In 1931, sulfur gave an open-minded Dutch-American graduate student, Cornelis B. van Niel and eventually the rest of the rest of the world new insight into photosynthesis. Purple sulfur bacteria found in salt marshes and mudflats produce organic matter in the presence of light, but they rely on hydrogen sulfide instead of water. The waste-product is not oxygen but sulfur.
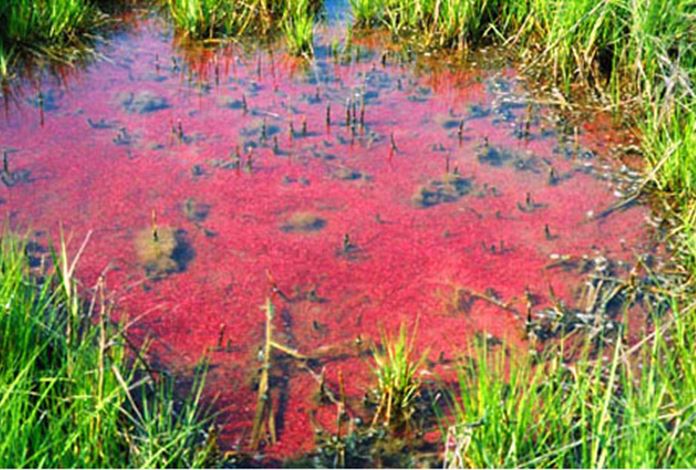
For the previous 100 years, scientists were under the false impression that in photosynthesis the entire water molecule was incorporated into sugars. They also incorrectly imagined that water effectively displaced oxygen from carbon dioxide. Seeing that purple sulfur bacteria(Figure 3) displaced sulfur from H2S, an analogue of H2O, Cornelius B. van Niel, correctly inferred that in plants, oxygen was displaced from water molecules.
Eventually more solid evidence supported van Niel’s hypothesis. Plants were given water that was made with the 18O isotope, a non-radioactive but heavier version of the common 16O isotope. They were grown in the atmosphere with the usual CO2, which has mostly 16O. When the oxygen produced by the plants was analyzed, it contained only 18O, proving that water is the source of oxygen gas.
iii. Sulfur from Io

The Hubble telescope was equipped with the Space Telescope Imaging Spectrograph (STIS) which revealed information about an object’s chemical content, temperature, density, and motion. Jupiter’s moon, Io, as any colored photograph hints(Figure 4), is covered with a thin coat of sulfur, which is emitted by its volcanoes. In 1999, STIS detected S2, a diatomic version of sulfur, above the volcano, Pele. S2 lands on Io’s cold surface, where sulfur atoms rearrange into tri- or tetra-atomic molecules (S3, S4). These lend a reddish color to the surface. Eventually the atoms rearrange into their most stable configuration, molecular rings of eight atoms (S8). This is the form that we see along the edges of cauldrons in Yellowstone Park. Interestingly, in the extraction of sulfur from heavy crude, sulfur is also initially in an unstable S2 form but then becomes S8, the form piling in the Fort McMurray pyramids and in the piles at the Vancouver port.
Sulfuric acid
In concentrated form, sulfuric acid, H2SO4, is a thick syrupy liquid. I recall using it while working in a quality control lab analysing the protein content of hot dogs. Despite the pressure differences of the fume hood which are meant to divert the acidic fumes away from the working environment, one of my colleagues became itchy all over her arms and torso. She reported to the company doctor but regretted her decision when she felt that he had taken advantage of her sensitive reaction to the acidic fumes. He had asked her to disrobe and expose her breasts. It was the first time in my young life that I had heard of unprofessional behaviour from an esteemed individual.
Industry’s most widely produced compound, H2SO4, is used in lead batteries, copper refining and especially in the production of phosphoric acid for phosphate fertilizers. It’s also needed to make other acids, pharmaceuticals and dyes. Currently, sulfuric acid is made industrially by burning sulfur and by catalytically oxidising the subsequent product (SO2) with vanadium(V) oxide. Contrary to many internet sources, the new product, SO3, is not added to water in the final step. That reaction is too exothermic for a large-scale operation. Instead SO3 reacts with previously made sulfuric acid to make H2S2O7, which then reacts with water to yield the acid.
When SO2 escapes into the environment, it becomes sulfuric acid through the reaction of hydroxyl radicals and moisture. This main component of acid rain has a serious impact on lungs, infrastructure and lakes. For this reason, industries equipped with the latest technology have scrubbers. Some of these use limewater to convert the SO2 into calcium sulfite and eventually into the useful CaSO4 · 2H2O, which is the material in wallboard.
If you are going to be a criminal, you don’t want to be a popular one or you will increase the likelihood of being held responsible for crimes that you did not commit. A great deal has been written about why onions make you cry, but the information contradicts. Is sulfuric acid being falsely “accused”? And how we’ll arrive at the truth might prove to be more fun than knowing the fact itself.
The 2012 chemistry textbook, General, Organic and Biological Chemistry, by H. Stephen Stokes; a PBS website on why onions make us cry, and chemistry.about.com all claim that, after the volatile lachrymator propanethial-S-oxide is produced by a cut onion’s enzymes, it reacts with water in the eyes to produce the irritant sulfuric acid. But Scientific American, Molecule of the Month and the analytical services of onionlabs.com attribute the lacrimatory effects directly to the molecule’s action on receptors. Sulfuric acid is not part of the explanation. From the latter:
“LF is the chemical compound which directly causes the eye to tear (often called the onion lachrymator) and the chemical sensation of heat or mouth burn when an onion is eaten. It is measured using HPGC equipment and is reported in µmoles of LF/ml of onion juice. . ..“
And then the details from a researcher writing in Scientific American:
“The cornea is densely populated with sensory fibers of the ciliary nerve, a branch of the massive trigeminal nerve that brings touch, temperature and pain sensations from the face and front of the head. The cornea also receives a smaller number of autonomic motor fibers that activate the lachrymal (tear) glands. Free nerve endings detect syn-propanethial-S-oxide on the cornea and drive activity in the ciliary nerve–which the central nervous system interprets as a burning sensation–in proportion to the compound’s concentration. This nerve activity reflexively activates the autonomic fibers, which then carry a signal back to the eye ordering the lachrymal glands to wash the irritant away.“
I started to get skeptical about the alleged formation of sulfuric acid for the following reason. If, in the presence of water in the eye, the lachrymator degrades so quickly, wouldn’t most of the propanethial-S-oxide break down in the onion’s aqueous environment? Let’s assume for a moment that some still escapes unscathed due to its relatively high vapor pressure of 41.2 ± 0.2 mm Hg at 25°C. To put that in perspective, water’s vapor pressure is only 23.8 mm Hg at that temperature, so the lachrymator is about as volatile as ethyl alcohol, whose vapor pressure is about 44 mm Hg.
To look for any pH-changes, I taped only the ends of a wet piece of litmus paper in a sealed plastic bag (Figure 5). I chopped an onion and quickly placed the pieces into a Ziploc, making sure that the acidic juices of the onion would not be in contact with the litmus. If the lachrymator indeed broke down into compounds that included sulfuric acid, then the exposed wet litmus should turn red between 30 seconds and couple of minutes, the time that it takes for the compound to form and reach the eyes. But there was no color change, consistent with the fact that the lachrymator itself has no pKa, a measure of acidic strength.
One could argue that the amount of acid produced was too small to affect the litmus. A paper on a rapid extraction method of the lachrymatory factor of onion found that each milliliter of onion juice contains 1−22 μmol of the tear-forming agent. Let’s assume a density of 1.0 g/ml and an average mass of 120 g per onion, which is about 90% water, and that about only 30% of the lachrymator reached half a ml of water on the litmus. If it had produced H2SO4 in a 1:1 ratio (only one source (the textbook) had included a chemical equation, and it was not even balanced), then we could easily calculate that we’d end up with a pH between 3 and 4, enough to turn the litmus red. I also mixed a small amount of vinegar with the onions, and within a few minutes, the same wet litmus did indeed turn red, revealing that the simple setup could detect acidity.
And one final detail, most literature on the topic including this blog so far, discuss the lachrymator as if it’s a single compound. But an NMR analysis in Tetrathedron Letters reveals it to be a 19 to 1 mixture of (Z)- and (E)-propanethial S-oxide (Figure 6).
These are not earth-shattering revelations. But what was humbling is how the inaccuracies from a field that I know and enjoy almost got past me. Imagine what happens when I read news about economics and terrorism, stories that I often do not dig into to uncover the truth.
Sulfate, the essential ion
Sulfate, SO42-, is a part of the sulfuric acid molecule, but if it’s bonded to a metal, the compound will not share acid’s most distinguishing properties. The sulfate ion itself does not burn skin or taste bitter. SO42- is also part of the planet’s natural sulfur cycle. Algae and plants absorb sulfate ion to make a pair of amino acids that contain sulfur, cysteine and methionine (Figure 7).
Humans cannot make their own methionine. Obtaining it from our diets, we ultimately rely on other organisms’ ability to do something with sulfate. Even when we make cysteine, we get the sulfur from methionine. The sulfur linkages between different cysteine molecules in a peptide chain help it fold and stabilise into a specific three-dimensional protein that define its role. The importance of shape and role can range from how curly your hair is to the proper functioning of enzymes in our cells, and without methionine no nucleated cell can even begin to make a protein. Bacteria in ruminants also use sulfate to produce cystine and methionine. This was discovered by using sulfate with a radioactively tagged isotope of sulfur.
Elsewhere sulfate is reduced to H2S by specialized bacteria while others can oxidize either H2S or elemental sulfur back to sulfate. Bear in mind that H2S is also produced by volcanoes along with sulfur dioxide, which can react in the atmosphere to yield sulfates again. Sulfate-containing particles are important in cloud formation; they serve as seeds for the condensation of water vapour.
If as a teacher or student you hear of an oxidation-reduction partnership, the words might conjure up an image of people partnering up to do a redox lab, which involves one substance losing electrons and the other sweeping them up. But until recently, not even the most specialized biochemists imagined that two different organisms were symbiotically working together to oxidize methane and reduce sulfate, respectively, in layers of sediment and rock under the sea floor. One of the microbes, a methanotroph which uses methane (CH4) as its energy and carbon source, reduces CH4 to hydrogen carbonate ion (HCO3–) in the absence of oxygen(Figure 8).
But the metal ions that the organism uses to pick up electrons lost by methane are not efficient enough. A sulfate-reducing bacteria comes to the rescue. It uses the electrons released by the oxidation of methane to reduce sulfate (SO42-) to sulfide (S2-). Its reward? It too obtains energy in the process.
Sodium sulfate is found in three forms: (1) Glauber’s salt (Figure 9) which is a hydrate of the salt(Na2SO4.10H2O); salt cake (mostly Glauber’s salt but mixed with magnesium sulfate and silica;and finally anhydrous sodium sulfate(Na2SO4). Sodium sulfate is needed to make brown paper, brown cardboard, glass, textiles and detergents. In 2016 China produced 60% of the world’s sodium sulfate and consumed 67% of the world’s share. Chaplin Lake in Saskatchewan, Canada has large natural reserves of salt cake, which is converted to 99.0% anhydrous sulfate, the so-called detergent-standard. To obtain that purity, the salt cake has to go through a log washer and then through a heating process that drives off the water from the hydrate.
Sulfur dioxide
SO2 is a useful gas in the production of sulfuric acid, but it is also an undesirable combustion product of coal, which is typically 1 – 4% sulfur. Nickel and copper ores are sulfur compounds, so in the roasting process, sulfur dioxide is again produced. Scrubbers are essential in filtering emissions and converting the smelly and lung-irritating gas to useful products. These were discussed in the sulfuric acid blurb.
Emission control systems are hindered by the presence of sulfur dioxide in exhaust. The catalyst in converters is designed to oxidize some of the carbon monoxide and dissociate some nitrogen oxides. SO2 remains intact and interferes with the catalyst. As a result, there has been pressure to remove more sulfur from transportation fuels. If the crude oil is originally sulfur-rich, it is dubbed “sour. Sweet crude oil contains less sulfur, and its combustion poisons less catalyst in converters and contributes less sulfur dioxide to the atmosphere.
Volcanoes are a natural source of sulfur dioxide. I once hiked near Kīlauea volcano in Hawaii and the SO2-containing volcanic smog was foul-smelling and made it difficult to breathe on the steeper parts of the hike. During major eruptions, SO2 can be injected to an altitude of over 10 km and into the stratosphere. There, SO2 is converted to sulfate aerosols which reflect sunlight and have a temporary cooling effect on the Earth’s climate. They also deplete ozone.
The overall volcanic contribution of SO2 to our atmosphere, however, pales in comparison to the amount we discharge. Emissions in North America and Europe peaked in the mid-70s but are currently at and of their maximum values, respectively. But meanwhile in east Asia, the emissions have reached Europe’s highest output from 40 years ago.
Sodium bisulfite (NaHSO3)
Sodium bisulfite, a source of the bisulfite ion, is made by reacting washing soda (sodium carbonate) with sulfur dioxide, the same starting point in the contact process-production of sulfuric acid. Water treatment plants use bisulfite to eliminate excess chlorine from both drinking water and treated sewage. Relative to chlorine, bisulfite acts as a reducing agent, allowing chlorine to gain electrons and become chloride. In a similar reaction, bisulfite can react with oxygen to prevent corrosion of large pipes.
An oxidation-reduction reaction involving bisulfite has a strong, peculiar smell that you can feel in your throat because the bisulfite ion and an acidic proton are in equilibrium with water and sulfur dioxide. Amateur wine makers use bisulfite as a reducing agent to prevent the oxidation and discoloration of white wine. They must be careful with concentrations; otherwise too much will leave a sulfurous taste that ruins the wine. Even when professionals are careful, they run up against individuals who are allergic to bisulfite, which makes them wheeze and develop hives.
Hydrogen sulfide (H2S)
The odour threshold for hydrogen sulfide gas is only 10 parts per billion to about 1.5 parts per million. It stinks like rotten eggs or like the nastiest type of flatulence, both of which involve the microbial breakdown of sulfurous amino acids into hydrogen sulfide. Luckily, we are sensitive to the smell because at 1 to 2 parts per thousand it causes instant death. In between the odor threshold and the lethal dose, it has unpleasant effects ranging from nausea, pulmonary edema and loss of consciousness. Since hydrogen sulfide is produced naturally from decaying organic matter, it can be released from sewage sludge and manure. Sulfur hot springs and natural gas are also sources. Some industrial processes that release it as a byproduct include wastewater treatment, petroleum refining and mining. Workers have died when ventilation and detection systems have been inadequate. Marriages have been threatened by the indiscretions of a partner suffering from H2S flatulence.
Let us not forget that one man’s poison is another organism’s lifeblood. Without H2S, purple sulfur bacteria would not be able to make sugars. It provides electrons and hydrogen ions that sustain their form of photosynthesis. In the South Andros Black Hole, Bahamas, a thick layer ofpurple sulfur bacteria lives at a depth of 17.8 m. In this thick layer which makes the water appear black at the surface, the temperature increases sharply to 36 oC as a result of the inefficient transfer of light from their accessory pigments, carotenoids, to their chlorophyll centers. This ability to raise the temperature of their immediate environment gives the mass of bacteria an advantage over other species that are incapable of the feat. And it’s all dependent on H2S.
Dimethyl sulfide
In both the chloroplasts and cytoplasm of phytoplankton, the sulfur-containing amino acid, methionine, is converted into dimethyl sulfonium propionate (DMSP). Why? DMSP is an organic salt that prevents water from leaving microscopic algal cells that live in the ocean’s salty environment, and at the same time DMSP does not interfere with protein function. The compound also helps bacteria that consume dead plankton.
Eventually, DMSP is broken down to acrylic acid and a vital component of the sulfur cycle known as dimethyl sulfide (DMS). It has been suggested that DMS and another compound known as isoprene are used by phytoplankton to mitigate harmful reactive oxygen species like H2O2 formed from dissolved oxygen, organic matter and iron stress.
Annually, approximately 28 × 1012 grams of sulfur are placed in the atmosphere when algae release the volatile DMS into the atmosphere. The compound is eventually oxidized to sulfate, which is an important cloud condensation nucleus. Although it hasn’t been substantiated by evidence, Charlson proposed a mechanism involving DMS that became part of Lovelock’s Gaia hypothesis. In this scenario, the abundance of cloud condensation nuclei from DMS-derived sulfate above the sea affects the amount of cloud cover, in turn affecting the radiation reaching the ocean. This feeds back into the climate, affecting marine biology and presumably regulating the amount of DMS produced by phytoplankton.
Sulfur compounds in minerals
This rock contains a pair of minerals, both of them sulfides: (1)the golden-colored pyrite a.k.a. fool’s gold ( iron sulfide), and sitting underneath is (2) sphalerite, which contains zinc sulfide the main ore of zinc. Galena (PbS, not the TV network but lead sulfide) is also found often together with sphalerite. |

Left, Elemental sulfur on aragonite , a mineral consisting of calcium carbonate. From Sicily. Right, Stibnite, which is mostly antimony sulfide, Sb2S3, is the principal ore of antimony. |
People like to include precious stones in jewelry. It is obviously not just about the stone; there is workmanship involved in adorning the stone; there is the association with status and enhancing the interest, if not beauty, of the person who owns and wears the mineral. But there is something more beautiful about minerals in their own natural state. Here is a look at those containing species of sulfur.
Gypsum contains calcium sulfate dihydrate, CaSO4·2H2O, the same mineral that is found in wallboard. As is the case with many minerals, gypsum’s color can vary depending on the impurities it contains. |
The colorless crystals consist of a sulfate of barium, known as barite. BaSO4 has a low solubility, preventing the otherwise poisonous barium from being released. The compound is opaque to x rays and in suspension, it is used in medicine for imaging the gastrointestinal track. Barium sulfate is also used in fireworks to create a green color. Source of sulfate mineral images: Wikipedia commons. |